MicroBooNE is the first large liquid-argon time projection chamber (LArTPC) to acquire a high statistics sample of neutrino interactions. With its superb capabilities in tracking, vertexing, calorimetry, and particle identification with full electronic readout, MicroBooNE represents a major advance in detector technology for neutrino physics in the energy regime of most importance for elucidating oscillation phenomena. These capabilities lead to objectives that combine immediate scientific interests with long-term technological innovations.
The experiment primary scientific objectives are to to resolve the source of the MiniBooNE low energy excess, to measure a suite of neutrino cross sections, to search for astrophysical phenomena and exotics. MicroBooNE is currently supernovae live, when a SNEWS alert is received, data will be saved to study neutrino bursts from supernova. Another important objective of MicroBooNE lies in the area of detector development. MicroBooNE will provide enormous technical experience in the construction, operation, and analysis of a large LArTPC. This experience will directly inform the design of very large LArTPC detectors envisioned for DUNE and beyond.
The MiniBooNE Low Energy Excess
The MiniBooNE experiment performed a short baseline search for the νμ→νe oscillation signature suggested by the Liquid Scintillator Neutrino Detector (LSND). MiniBooNE observed an unexpected >4σ (statistical and systematic combined) excess of neutrino interactions producing final state electrons or photons at lower energies. This result generated substantial theoretical interest. Proposed explanations for the source of these events predict either a single electron track or a single photon produced at the neutrino interaction vertex. MiniBooNE cannot discriminate between these two possibilities, as Cherenkov detectors cannot distinguish an electron from a photon converting near the interaction point.
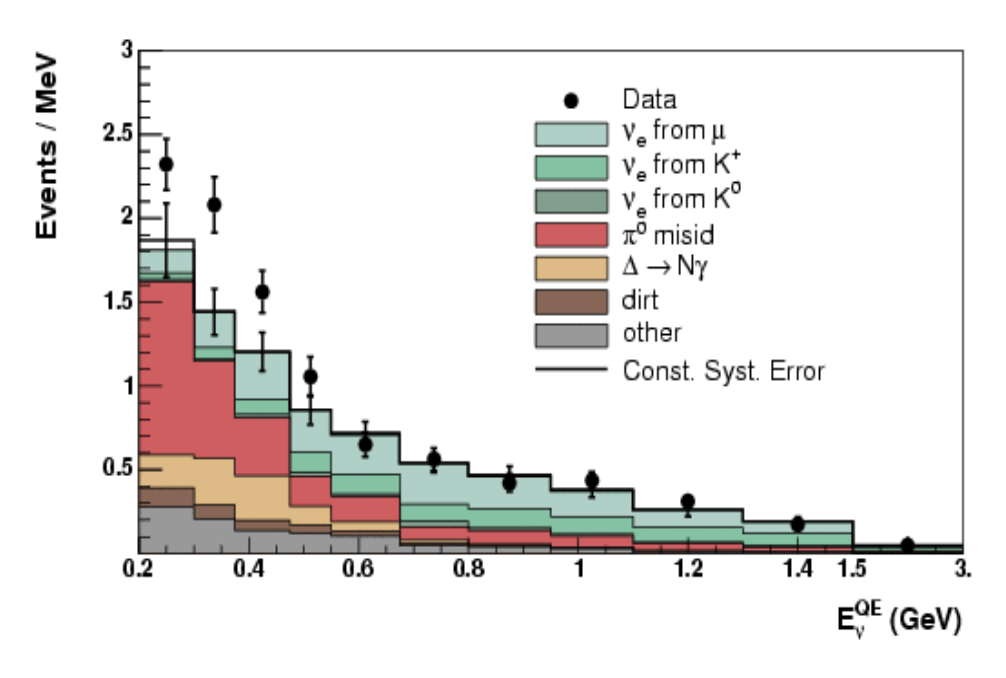
MiniBooNE low energy excess in neutrino mode. Backgrounds are shown in the stacked histogram and come from νe events, which are electron-like, and also misidentified νμ events, which are photon-like
Understanding the source of the MiniBooNE excess is MicroBooNE’s primary physics goal. With the excellent capabilites of the MicroBooNE Liquid Argon Time Projection Chamber (TPC) detector, MicroBooNE can resolve individual particle tracks including differentiating electrons from photons.
The MicroBooNE analysis proceeds in two modes, one that assumes that an electron interaction produces the signal (electron-like analysis) and the other that assumes a photon interaction generates the signal (photon-like analysis). Electrons distinguish themselves from photon conversions to an e+e– pair by their different apparent ionization rate at the beginning of their trajectory. Put simply, an e+e– pair ionizes at twice the rate of a single electron.
Cross Section Measurements
MicroBooNE has collected the world’s largest dataset of neutrino-argon interactions and is pursuing a broad program of cross-section measurements. These analyses leverage LArTPC detector technology to examine neutrino scattering physics in great detail. Strengths of the MicroBooNE detector for pursuing these results include
- Excellent efficiency for reconstructing low-energy activity;
- Strong capabilities for distinguishing between different particle types;
- High resolution for spatial event reconstruction and measurements of proton energies and scattering angles;
- Sophisticated methods for observing and reconstructing complicated event topologies;
- Long-term exposure to both Fermilab neutrino beamlines (BNB and NuMI).
These advantages are enabling MicroBooNE to deliver pioneering interaction measurements that serve as critical input to the ongoing worldwide effort to answer fundamental questions related to the physics of neutrinos. Some of the neutrino cross sections which have been measured recently by the collaboration are
- Total and differential νe + v̅e charged-current inclusive
- Pionless νμ charged-current with one or more final-state protons
- Quasielastic-like νμ charged-current
- Neutral pion production via νμ charged-current
- Charged-particle multiplicity and double-differential νμ charged-current inclusive
Many more cross-section analyses are currently underway within the MicroBooNE collaboration. A few examples of topics under investigation include
- Neutral-current elastic
- Pion production via charged- and neutral-current channels
- Hadronic and neutrino energy reconstruction in νμ charged-current
- Proton-containing final states for νμ and νe charged-current
- Hyperon, kaon, and η meson production
Supernova Detection and Proton Decay Background Measurements
MicroBooNE surface location limits its palette of non-accelerator neutrino physics topics. This siting reflects a conscious design decision to focus on beam related physics, and not to expand the design in a substantial way to incorporate non-accelerator physics.
One exciting non-accelerator physics topic open to the experiment with only modest expansion to its design is supernova (SN) detection through a short pulse of neutrino interactions observed in the detector. If a supernova were to occur in our galaxy, MicroBooNE would detect more events than were observed, in total, from SN 1987a. Moreover, because these SN events produce electrons in the >10 MeV range, the design requirements on MicroBooNE do not substantially extend beyond those already in place for collecting a substantial muon decay electron sample for energy calibration studies. In the process of seeking this physics, MicroBooNE addresses several technical issues for future detectors.